ThermoChemical and Thermal
Scanning Probe Lithography tc- and t-SPL
Progress in nanotechnology depends on the capability to fabricate, position, and interconnect nanometer-scale structures. Thermochemical nanolithography, TCNL, now called tc-SPL or t-SPL was invented in Riedo's laboratory at Georgia Tech in 2007 [32, 4]. TCNL uses a localized source of heat to activate chemical reactions at the macro-down-to the atomic scale. We use thermal AFM cantilevers with variable temperature tips to implement TCNL for a variety of applications in biology, nanomedicine, nanoelectronics, and nanophotonics.
So far, we have demonstrated the ability of TCNL to generate proteins arrays with 10 nm-resolution [1-32], and concentration gradients of amines and proteins with sub-100 nm resolution [2, 24]. TCNL was also implemented to fabricate conjugate semiconducting polymer nanowires [21], piezoelectric/ferroelectric PZT and PTO ceramic nanostructures [14] and graphene ribbons [20].
Clearly, TCNL has a broad range of applicability given the extreme writing speed (mm/s for most of the above mentioned reactions), potential massive parallelization (use of tip array has been demonstrated [10]), and high resolution (down to 10 nm),
At present our research program on TCNL is following different directions, in particular:
(i) 2D Materials. Recently, there has been enormous progress in the production of atomic crystals that are strictly two dimensional (2D) from graphene, to h-BN, and transition metal dichalcogenides (TMD). My group is particularly interested in the possibility to tune the properties of 2D materials locally by means of ad-hoc chemical functionalization [9, 15]. The goal is to use TCNL to thermally control the chemistry of 2D materials, and therefore their properties. I develop these studies in collaboration with other groups for sample growth, and theory. Right now, we are concentrating our efforts in understanding the reduction of functionalized graphene, and Mo-oxide, as well as the oxidation of MoS2 and other TMD.
(ii) Biomedical Applications. So far, we have proved that TCNL can be used to change with nanoscopic resolution the chemistry of ad-hoc polymer films to expose amine groups, and carboxylic acid groups, and subsequently to functionalize these nanopatterns with different types of nano-objects, such as DNA, proteins, and C60 [2, 24]. TCNL can therefore be used to prepare with 10 nm resolution either proteins and peptides patters, or chemical concentration gradients. In particular, interesting opportunities could arise in patterning electroconducting gels to study neurons growth, stem cells differentiations and neuron sub-cellular activity.
(iii) Metamaterials and Spintronics Devices. TCNL and other tip-based nanofabrication techniques coupled with more conventional lithography methods could be employed for the fabrications of optical and magnetic structures for magneto-plasmonic and spinwaves applications. In particular, we have very recently demonstrated that TCNL can craft at the nanoscale the magnetic anisotropy landscape of a ferromagnetic layer exchange-coupled to an antiferromagnetic one [1]. By performing a highly localized field cooling by means of TCNL, unconventional magnetic domains, with arbitrarily oriented magnetization and tunable unidirectional anisotropy, are patterned without modifying the substrate topography. This discovery allows an unprecedented non-destructive control over the magnetic properties of thin films, and opens unforeseen possibilities for the development of metamatarials for reconfigurable multiple state (beyond binary) memory, logic and sensing magnetic devices architectures. This work is currently developed in collaboration with the Politecnico of Milano.
(iv) 2D transistors. Two-dimensional semiconductors, such as molybdenum disulfide (MoS2 ), exhibit a variety of properties that could be useful in the development of novel electronic devices. However, nanopatterning metal electrodes on such atomic layers, which is typically achieved using electron beam lithography, is currently problematic, leading to non-ohmic contacts and high Schottky barriers. Recently, we show that thermal scanning probe lithography can be used to pattern metal electrodes with high reproducibility, sub-10 nm resolution, and high throughput (10^5 μm^2/h per single probe). The approach, which offers simultaneous in situ imaging and patterning, does not require a vacuum, high energy, or charged beams, in contrast to electron beam lithography. Using this technique, we pattern metal electrodes in direct contact with monolayer MoS2 for top-gate and back-gate field-effect transistors. These devices exhibit vanishing Schottky barrier heights (around 0 meV), on/off ratios of 10^10, no hysteresis, and subthreshold swings as low as 64 mV per decade without using negative capacitors or heterostacks. Our results show that t-SPL is compatible with standard etching procedures and could potentially be pushed to single-digit-nm spatial resolution, and, by multiplexing with thermal nanoprobe arrays, to higher throughput. The approach could thus lead to low-cost, no-vacuum, one-step industrial metal nanomanufacturing.
References:
1. Xiaorui Zheng, Annalisa Calò, Edoardo Albisetti, Xiangyu Liu, Abdullah Sanad M. Alharbi, Ghidewon Arefe, Xiaochi Liu, Martin Spieser, Won Jong Yoo, Takashi Taniguchi, Kenji Watanabe, Carmela Aruta, Alberto Ciarrocchi, Andras Kis, Brian S. Lee, Michal Lipson, James Hone,Davood Shahrjerdi, Elisa Riedo* "Patterning metal contacts on monolayer MoS2 with vanishing Schottky barrier via thermal nanolithography" Nature Electronics 2, 17–25 (2019), doi: https://doi.org/10.1038/s41928-018-0191-0
2. Xiangyu Liu, Mohit Kumar, Annalisa Calo’, Edoardo Albisetti, Xiaouri Zheng, Kylie B. Manning, Elizabeth Elacqua, Marcus Weck, Rein V. Ulijn, Elisa Riedo*, "High-throughput Enzyme Nanopatterning", Faraday Discussion, (2019). doi: 10.1039/C9FD00025A
3. E. Albisetti, D. Petti, M. Pancaldi, M. Madami, S. Tacchi, J. Curtis, W.P. King, A. Papp, G. Csaba, W.Porod, P. Vavassori, E. Riedo, R. Bertacco, “Nanopatterning reconfigurable magnetic landscapes via thermally assisted scanning probe lithography” Nature Nanotechnology, (2016) doi:10.1038/nnano.2016.25 (Cover article)
4. Albisetti, Edoardo; Carroll, Keith; Xi, Lu; Curtis, Jennifer; Petti, Daniela; Bertacco, Riccardo; Riedo, Elisa, "Thermochemical scanning probe lithography of protein gradients at the nanoscale" Nanotechnology, (2016) Article reference: NANO-110170.R2.
5. Abdelghani Laraoui, Halley Aycock-Rizzo, Yang Gao, Xi Lu, Elisa Riedo, Carlos Meriles, “Imaging thermal conductivity with nanoscale resolution using a scanning spin probe” Nature Communications 6, 8954, (2015) doi:10.1038/ncomms9954
6. Ricardo Garcia, Armin Knoll, and Elisa Riedo “Advanced Scanning Probe Lithography”, Nature Nanotechnology, 9, 577 (2014) DOI: 10.1038/NNANO.2014.157.
7. Keith M. Carroll, Maitri Desai, Anthony J. Giordano, Jan Scrimgeour, William P. King, Elisa Riedo, and Jennifer E. Curtis “Speed Dependence of Thermochemical Nanolithography for Gray-Scale Patterning”, in press in ChemPhysChem, (2014) DOI: 10.1002/cphc.201402168.
8. Keith M. Carroll, Xi Lu, Suenne Kim, Yang Gao, Hoe-Joon Kim, Suhas Somnath, Laura Polloni, Roman Sordan, William P. King, Jennifer E. Curtis, Elisa Riedo “Parallelization of Thermochemical Nanolithography”, Nanoscale 6 (3), 1299 – 1304, (2014).
9. K. M. Carroll, A. J. Giordano, D. Wang, V. K. Kodali, J. Scrimgeour, W. P. King, S. R. Marder, E. Riedo, and J. E. Curtis, “Fabricating nanoscale chemical gradients with thermochemical nanolithography,” Langmuir, 29 (27), 8675–8682 (2013).
10. Suenne Kim, Yaser Bastani, Haidong Lu, William P. King, Seth Marder, Kenneth H. Sandhage, Alexei Gruverman, Elisa Riedo, and Nazanin Bassiri-Gharb “Direct fabrication of arbitrary-shaped ferroelectric nanostructures on plastic, glass and silicon substrates”, Adv. Mat., 23, 3786–3790, (2011). (Cover Article)
11. Z. Q. Wei*, D. B. Wang*, S. Kim, S. Y. Kim, Y. K. Hu, M. K. Yakes, A. R. Laracuente, Z. T. Dai, S. R. Marder, C. Berger, W. P. King, W. A. de Heer, P. E. Sheehan, and E. Riedo, "Nanoscale Tunable Reduction of Graphene Oxide for Graphene Electronics," Science, 328, 1373-1376, (2010). **These authors contributed equally to this work.
12. D. B. Wang, S. Kim, W. D. Underwood, Lee, W. P. King, R. Marder, E. Riedo, “Direct Writing and characterization of PPV nanostructures”, Appl. Phys. Lett. 95, 233108 (2009).
13. D. Wang, V. Kodali, W. D. Underwood, J. E. Jarvholm, T. Odaka, S. C. Jones, M. Rumi, Z. Dai, W. P. King, S. R. Marder, J. E. Curtis, and E. Riedo “Thermochemical nanolithography of multi-functional templates for assembling nano-objects” Adv. Funct. Mat. 19, 3696 (2009) (Cover Article).
14. E. Gnecco, E. Riedo, W.P. King, S.R. Marder and R. Szoszkiewicz, “Linear ripples and traveling circular ripples produced on polymers by thermal AFM probes” Phys. Rev. B 79, 235421 (2009).
15. D. B. Wang, M. Lucas, R. Szoszkiewicz, E. Riedo, T. Okada, S. C. Jones, S. R. Marder, Lee, W. P. King, “Local wettability modification by thermochemical nanolithography with write-read-overwrite capability”, Appl. Phys. Lett. 91, 243104 (2007) (Highlighted by Virtual Journal of Nanoscale Science & Technology).
16. R. Szoszkiewicz, T. Okada, S. C. Jones, T.-D. Li, W. P. King, S. R. Marder and E. Riedo “High-speed, thermochemical nanolithography with sub-15 nm feature size”, Nano Letters 7, 1064 (2007). (Highlighted by Scientific American, UPI, and other News Media).
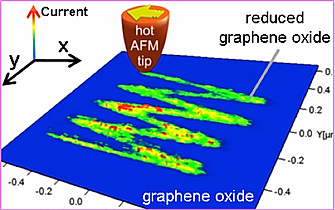
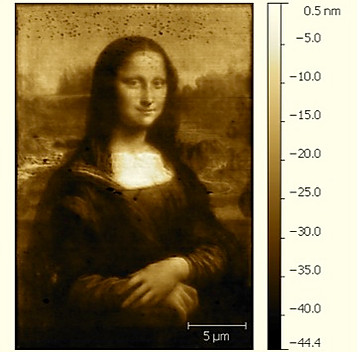

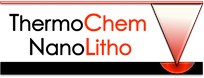
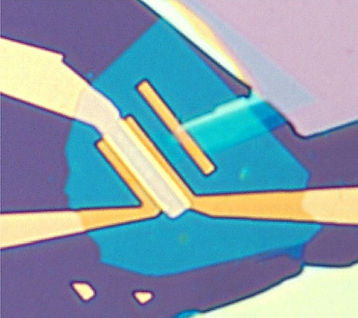